Battery Chemistry Basics
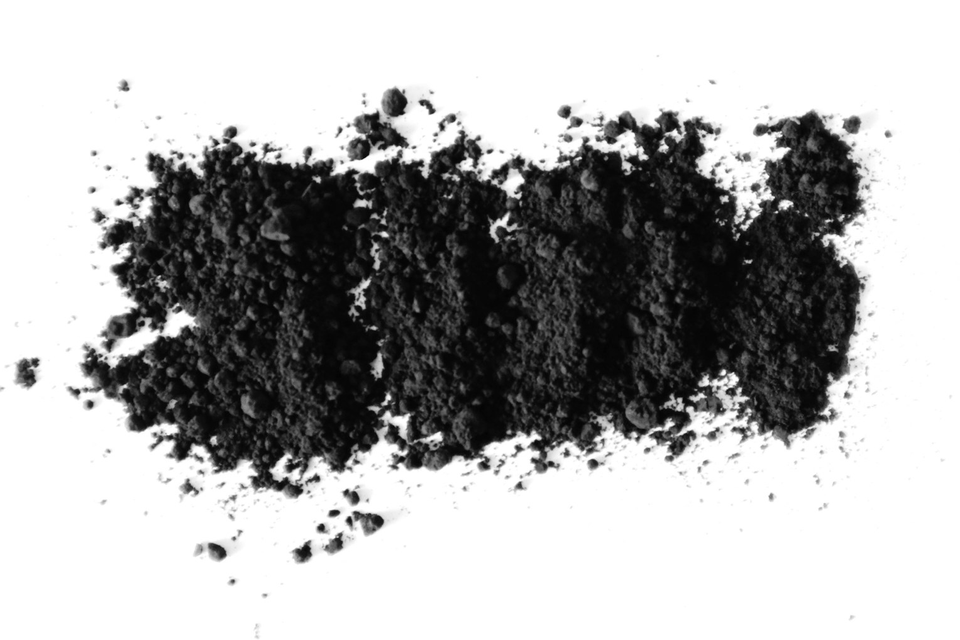
Introduction:
Before getting too far into the details of electrode manufacturing it is important to understand a bit more about the chemistries to be processed. In general, both cathode and anode electrodes are processed on similar equipment starting with slurry mixing followed by coating and drying. Future articles will delve into each unit operation in more detail but for now we will set the foundation and focus on common chemistries in use today.
In the pursuit of sustainable energy solutions, lithium-ion batteries have emerged as a pivotal technology driving the transition towards cleaner energy sources. From powering our smartphones to revolutionizing electric vehicles (EVs), lithium-ion batteries have become the cornerstone of modern energy storage. However, to fully harness their potential and accelerate the shift towards a greener future, it is imperative to delve deeper into the chemistry behind these batteries.
Understanding Lithium-Ion Battery Chemistry:
At the heart of every lithium-ion battery lies a complex interplay of chemical reactions. The fundamental principle revolves around the movement of lithium ions between the cell's positive (cathode) and negative (anode) electrodes during charge and discharge cycles. This process is facilitated by the migration of lithium ions through an electrolyte solution, typically composed of lithium salts dissolved in a solvent. Also included in this assembly is a thin porous membrane or separator film that insulates the cathode and anode from each other, designed to prevent an internal short circuit.
Cathode: The cathode is typically constructed from lithium metal oxides such as lithium cobalt oxide (LiCoO2), lithium iron phosphate (LiFePO4), or lithium nickel manganese cobalt oxide (NMC). These materials play a crucial role in determining the battery's energy density, voltage, and cycle life. Advancements in cathode chemistry have led to the development of high-capacity materials capable of storing more energy per unit weight. The radar plots below provide an overview comparing commercially available cathode materials.
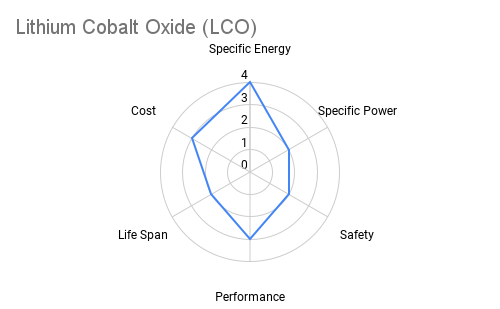
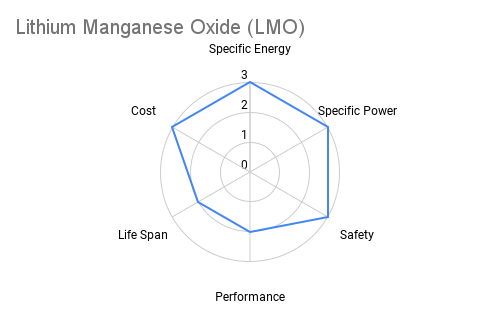
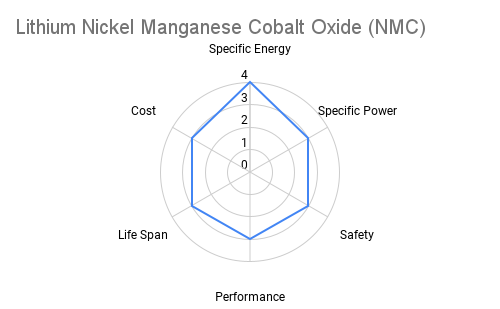
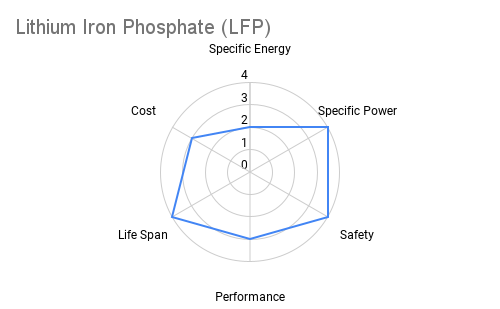
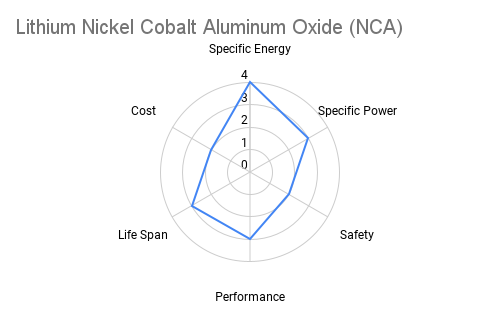
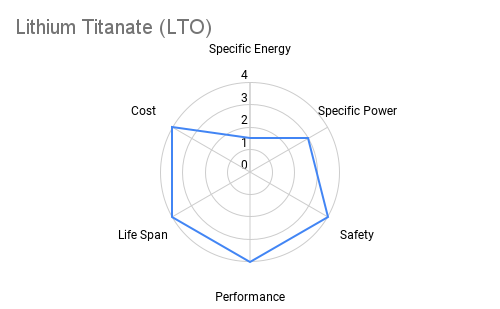
Furthermore the following table provides some additional context on the advantages and applications each cathode chemistry offers.
Cathode Material | Advantages | Applications |
---|---|---|
LiCoO2 (LCO) | High specific energy, good cyclability | Consumer electronics, mobile phones, laptops, cameras |
Lithium Manganese Oxide (LMO) | High power density, good thermal stability | Medical devices, hybrid and EV's, power tools |
Lithium Nickel Manganese Cobalt Oxide (NMC) | Low cost, high energy density | Medical devices, EV's, E-bikes |
LeFePO4 (LFP) | Low cost, long cycle life | Stationary storage, industrial, renewed focus on EV's |
LiNixCoyAlzO2 (NCA) | Low cost, high energy density | EV's, other electrical powertrains |
Lithium Titanate (LTO) | High safety, fast charge, wide temperature range | Aerospace, military, EV's, telecomm., UPS |
Anode: Traditional anode materials, such as graphite, have been widely used in lithium-ion batteries due to their stability and abundance. However, researchers are exploring alternative materials, including silicon and lithium metal, to enhance the battery's energy density and cycling stability. Silicon, in particular, has garnered attention for its high theoretical capacity, although challenges related to volume expansion during lithiation remain a hurdle to overcome.
Fabrication of the cathode and anode electrodes are at the core of Electrode School. Each chemistry poses new or different challenges to the electrode manufacturing process. In later articles we will delve deeper into those challenges and opportunities for improvement.
Electrolyte: The electrolyte serves as a medium for lithium ion transport between the cathode and anode. Commonly used electrolytes include organic solvents such as ethylene carbonate and propylene carbonate, combined with lithium salts such as lithium hexafluorophosphate (LiPF6). The choice of electrolyte composition significantly influences the battery's performance, safety, and operating temperature range.
Persuading Towards Progress:
As a consumer and a participant in the energy storage market, I advocate for continued research and innovation to address the key challenges facing this technology and the ramp up of manufacturing capacity. One of the primary concerns is the reliance on scarce and geopolitically sensitive materials such as cobalt. By developing cathode materials with reduced cobalt content or exploring cobalt-free alternatives, the energy storage market can mitigate supply chain risks and promote sustainability. That said, new chemistries do pose new or different challenges in manufacturing. Cell manufacturers have to balance specialized process equipment with enough flexibility to adapt to new chemistries without significant process development costs or an increase in quality related defects.
Moreover, enhancing the energy density and cycle life of lithium-ion batteries is essential for expanding their applications beyond consumer electronics and EVs. This involves optimizing electrode materials, electrolyte formulations, and cell designs to achieve higher energy storage capacities and prolonged lifespan. Innovation in materials science, coupled with computational modeling and advanced manufacturing data analytics, offer promising avenues for accelerating this progress.
Furthermore, improving the safety of lithium-ion batteries is paramount to instilling confidence among consumers and fostering widespread adoption. Strategies such as incorporating flame-retardant additives, designing robust cell structures, and implementing advanced battery management systems (BMS) can mitigate the risk of thermal runaway and catastrophic failure.
Looking Ahead:
As we stand at the forefront of a renewable energy revolution, batteries hold the key to unlocking a sustainable future. By harnessing the power of chemistry and embracing a collaborative approach, we can overcome the technical barriers hindering widespread adoption and pave the way for a cleaner, greener tomorrow.
In conclusion, lithium-ion battery chemistry serves as the backbone of modern energy storage solutions, powering everything from portable electronics to electric vehicles and distributed energy storage. By understanding the intricate interplay of components and processes involved, we can appreciate the remarkable efficiency and versatility of lithium-ion batteries. As we continue to push the boundaries of innovation in battery technology, the future holds boundless possibilities for cleaner, more sustainable energy solutions.
We hope you found this exploration of lithium-ion battery chemistry informative and engaging. Do you have any questions or insights to share? Drop us a line, we'd love to hear from you. Also, subscribe to get future articles sent directly to your inbox!